Yong Ding, Xiangke Lv, Min Zhang,
Xiaodong Zhu, Qingzhong Yang
Ningbo Yunsheng Co., Ltd.
Rare earth element (REE) based Nd-Fe-B magnets have been widely used because of their excellent magnetic properties. The applications of Nd-Fe-B rare earth permanent magnets include hybrid electric vehicles (HEVs), power generators for wind turbines, high performance industrial motors, hard disk drives (HDD) for computers, traveling wave tubes for satellite communications, headphones and speakers, washing machines, and other consumer electronic devices. Furthermore, as well known, Dy and Tb are used as an additive to Nd-Fe-B magnets to increase the coercivity (because they increase the magneto-crystalline anisotropy) of magnets. But the magnetic moment of Dy and Tb are coupled anti-parallel to that of Fe, which decrease the magnetization and maximum energy product of the magnet. In addition, due to pressures on the supplies and prices of the heavy rare earth elements, recently there has been a drive towards less consumption of these materials for magnets. The grain boundary diffusion (GBD) has become one of the basic production routes to produce Nd-Fe-B permanent magnets with high performance, using less heavy rare earth. In the grain boundary diffusion process, heavy rare earth elements (HREs) such as Dy or Tb was penetrated preferentially along the grain boundaries rather than alloying into the entire grains. This results a dramatic improvement in coercivity, without losing remanence, leading to substantial savings in HREs. The material containing the heavy rare-earth elements applied in GBD process, can be in the form of metal elements[1-3], oxides[4,5], fluorides[4,6,7] and low-melting point eutectic alloys[8,9]. In this article, the mechanism and effect on the magnetic properties of TbH3 plus Tb4O7 mixture GBD process on sintered Nd-Fe-B magnets were investigated compared to Tb4O7 GBD process.
Experimental
A commercial sintered Nd-Fe-B magnet with a nominal composition of (NdPr)27.5Tb3Dy1.5Co0.5Ga0.15Cu0.1Al0.1Zr0.15Fe66.06B0.96 (numbers are related to weight percent) was used as the original material for GBD treatment. Firstly, the original magnet was washed with 3 percent HNO3 solution for 1 minute Then, the cylinder samples with the size of Φ10 by 2 mm3, Φ10 by 4.5 mm3, Φ10 by 7 mm3 with axial magnetization were coated with Tb4O7 or TbHx plus Tb4O7 mixture. Both groups contained the same amount of Tb in the coating layer. These coated magnets were exposed to a diffusion treatment at 900°C for 10 hours, followed by annealing at 500°C for 4 hours. The whole process was under protection of high vacuum. The room temperature magnetic properties of the samples were measured by pulsed field magnetometer (PFM, Hirst Magnetic Instruments LTD, PFM14.CN). The microstructure and elemental distributions inside the magnets were observed by Electron probe microanalyzer (EPMA, JEOL, JXA-8230). And the content of each element of magnets were tested by ICP (VARIAN, 725-ES). X-ray diffraction (XRD) analysis of the magnet was conducted using Cu Kα radiation with a Bruker D8 Discover X-ray diffractometer.
Results and Discussion
Figure 1 shows the 4πM-H and B-H demagnetization curves of the magnet before (sample A), and after GBD-treatment (only Tb4O7 sample B; TbH3 plus Tb4O7 sample C). The Hcj of Tb4O7 GBD treated magnet increases from 29.26 kOe to 35.17 kOe with only 0.05 kGs reduction of Br. For TbH3 plus Tb4O7, Hcj increases further to 37.17 kOe while Br is reduced by 0.08 kGs.
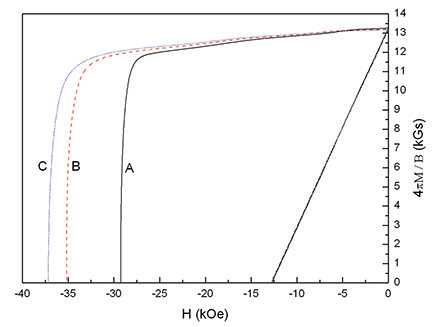
For GBD treatment, Tb diffuses from the surface into the inside of magnet. Tb causes a higher magneto-crystalline anisotropy field, which leads to the increase of Hcj. In order to investigate the effect of the addition of TbH3, the distribution of the Tb was tested by EPMA. Figure 2 shows the distribution of Tb over the cross section of Tb4O7 GBD treated magnet (sample B) and TbH3 plus Tb4O7 mixture GBD treated magnet (sample C). From the EPMA results, it is found that the diffusion depth is obviously different; the Tb diffuses deeper using the TbH3 plus Tb4O7 coating, which means more Tb diffuses into the magnet for TbH3 plus Tb4O7 mixture GBD treated magnet.

As shown in Table 1, the ICP results show the same result. The content of Tb in TbH3 plus Tb4O7 mixture GBD treated magnet is more than that in Tb4O7 GBD treated magnet. This indicates the addition of TbH3 can effectively enhance the diffusion of Tb. TbH3 will be decomposed into hydrogen and Tb during heat treatment process[10], the hydrogen can optimize diffusion atmosphere and enhance diffusion effect.

XRD patterns of GBD magnet are shown in Figure 3. As the magnet has been oriented, which means the magnet is anisotropic, only some XRD peaks of the 2:14:1 phase is obtained. In comparison to the XRD results from the centre of magnet, the XRD peaks of the surface grains shift to higher angle degree. This means crystal lattice constant of 2:14:1 phase changed with diffusion depth. As shown in Figure 3, the distance of the (006) peak of 2:14:1 phase in the surface layer is smaller than that in the centre area of magnet. This indicates that Tb diffuses into the 2:14:1 phase from the grain boundary. (Nd,Tb)2Fe14B phase will be formed in the surface region of the matrix grains after GBD. Bae et al. found the similar results[11], core-shell microstructure was formed in sintered magnets doped with Dy2O3 and DyHx powders. This enhances the magneto-crystalline anisotropy field of the matrix grains remarkably.
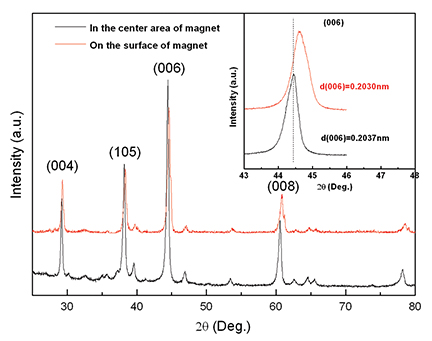
According to the above research results of grain boundary diffusion (GBD) technique, TbH3 plus Tb4O7 mixture GBD treatment is more superiority for magnetic property enhancement. So it is worth to investigate the details of GBD technique with TbH3 plus Tb4O7 mixture.
Figure 4 shows the relation between the thickness of a magnet and its properties when using the same amount of TbH3 plus Tb4O7 mixture per surface area. Sample size was Φ10 by 2 mm3, Φ10 by 4.5 mm3 and Φ10 by 7 mm3. As the magnet becomes thicker, Br goes up, Hcj and (BH)max+Hcj go down. Figure 4 shows that the specific surface area and Hcj have the same trend. So the specific surface area is the key element which affects the magnet properties. The larger the specific surface area is, the more Tb diffuses into the magnet, the higher Hcj, the smaller Br.
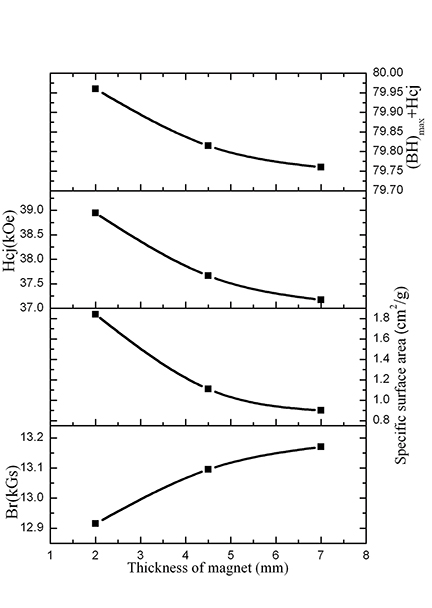
magnetic properties for GBD magnet (Φ10 by 2 mm3, Φ10 by 4.5 mm3, Φ10 by 7 mm3).
Figure 5 shows the relationship between the magnetic properties and the weight of TbH3 plus Tb4O7 coating (Φ10 by 4.5 mm3). When the weight is less than 0.03 g, then Br and (BH)max are almost unchanged but Hcj increases rapidly from 29.41 kOe to 37.67 kOe. Due to the impact of Hcj, the change trend of (BH)max+Hcj is the same as that of Hcj. When the weight of coating material increase from 0.03 g to 0.08 g, more Tb penetrates into the magnet, however Hcj no longer increases, Br and (BH)max decrease slightly firstly and then decrease significantly. Due to the combined effects of Hcj and (BH)max, there is a small decline of the (BH)max+Hcj. In summary, when the weight of TbH3 plus Tb4O7 mixture coating on the surface of magnet is between 0.03 g to 0.05 g, the GBD magnet has the best magnetic property, and the maximum of (BH)max+Hcj.

By optimized GBD process, a magnet with remarkably high performance has been achieved with intrinsic coercivity, Hcj, at 38.38 kOe and maximum energy product, (BH)max, at 40.39MGOe at 20°C. They give (BH)max (MGOe) + Hcj (kOe) = 78.77 (Figure 6).
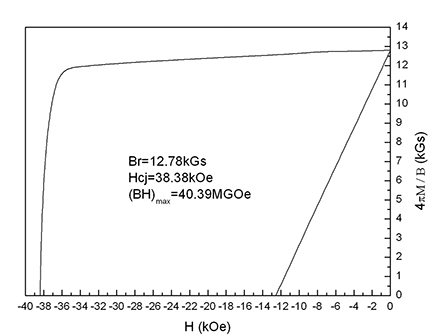
Conclusion
In this work, we investigated the microstructure and mechanism of coercivity enhancement of sintered Nd-Fe-B magnet prepared by grain boundary diffusion (GBD) technique with TbH3 plus Tb4O7 mixture coating. It is found that the intrinsic coercivity, Hcj, of magnet from above technique is much larger than that from Tb4O7 GBD treatment. The EPMA and ICP results indicate that the addition of TbH3 can effectively enhance the diffusion of Tb.
The XRD analysis results show that crystal lattice constant of 2:14:1 phase changed with diffusion depth. Because Tb diffuses into the 2:14:1 phase from the grain boundary phase, the crystal lattice constant of 2:14:1 phase in the surface layer become smaller than the constant in the center area of magnet.
The details of the GBD process using TbH3 plus Tb4O7 mixture were also studied. For the cylinder magnet with dimensions of Φ10 by 4.5 mm3, when using TbH3 plus Tb4O7 mixture as coating material and coating weight is between 0.03g/cm2 to 0.05g/cm2, the GBD magnet has the best magnetic property. By optimized GBD process, the magnet with (BH)max (MGOe) + Hcj (kOe) =78.77 was obtained.
Acknowledgement
This work was supported by the National High-Tech Research and Development Program of China (863 Program). This work was also supported by Ningbo Yunsheng Co., Ltd.
References
[1] H. Sepehri-Amin, T. Ohkubo, K. Hono, J. Appl. Phys. 107 (2010) 09A745.
[2] N. Watanabe, H. Uemoto, M. Ishimaru, M. Itakura, M. Nishida, K. Machida, J. Microsc. 236 (2009) 104-108.
[3] N. Watanabe, M. Itakura, N. Kuwano, D. Li, S. Suzuki, K. Machida, Mater. Trans. 48 (2007) 915-918.
[4] H. Nakamura, K. Hirota, M. Shimao, T. Minowa, M. Honshima, IEEE Trans. Magn. 41 (2005) 3844-3846.
[5] X.X. Sun, X.Q. Gao, M.C. Zhang, Q.F. Dong, S.Z. Zhou, J. Chinese Rare Earth Soc. 27 (2009) 86-92.
[6] K. Hirota, H. Nakamura, T. Minowa, M. Honshima, IEEE Trans. Magn. 42 (2006) 2909-2911.
[7] H. Suzuki, Y. Satsu, M. Komuro, J. Appl. Phys. 105 (2009) 07A734.
[8] N. Oono, M. Sagawa, R. Kasada, H. Matsui, A. Kimura, J. Magn. Magn. Mater. 323 (2011) 297-300.
[9] H. Sepehri-Amin, T. Ohkubo, T. Nishiuchi, S. Hirosawa, K. Hono, Scr. Mater. 63 (2010) 1124-1127.
[10] V.A. Yartys, O. Gutfleisch, V.V. Panasyuk, I.R. Harris, J. Alloys Compd. 253-254 (1997) 128–133.
[11] Kyoung-Hoon Bae, Tae-Hoon Kim, Seong-Rae Lee, Seok Namkung, and Tae-Suk Jang, J. Appl. Phys. 112 (2012) 093912.
For more information, visit www.yunshengusa.com.