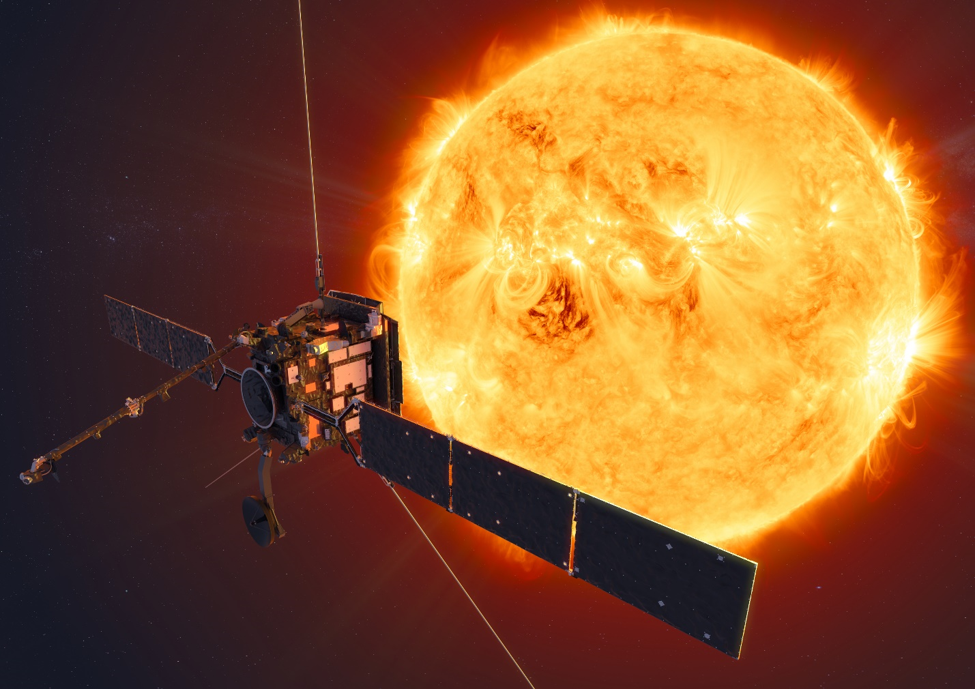
Magnetics technology is flying high, hot and cold in the European Space Agency’s Solar Orbiter mission to the sun. An extremely sensitive magnetometer built by Imperial College of London is a central element of the journey. A pair of specially configured Neodymium permanent-magnet micromotors from Maxon are also onboard playing a crucial supporting role, and a magnetometer from a French research agency will help another instrument characterize heating of the turbulent solar wind.
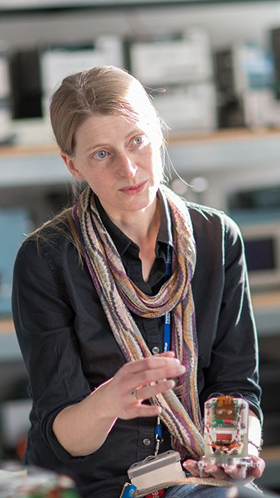
Launched February 10, Solar Orbiter will remotely measure the polar magnetic fields of the Sun for the first time. Imperial’s magnetometer, one of 10 instruments aboard, was the first to turn on, sending back data that shows it working even better than expected. “We are delighted with the measurements and the performance of the instrument, it looks even better than we hoped it would,” reported Helen O’Brien of Imperial, the instrument manager.
It is designed to help answer one of the top science questions to be explored by Solar Orbiter. The Sun’s magnetic field dominates the solar atmosphere. It produces all the observed energetic phenomena and displays an 11-year activity cycle but details of the so-called “solar dynamo” processes that power the Sun’s magnetic activity cycle are not yet fully understood.
The magnetometer will play a key role in understanding how the magnetic field links into the interplanetary medium and how long-term proxy records can be signatures for changes in solar conditions. Solar wind fills the solar system with charged particles and the Sun’s magnetic field, which can interact with the Earth’s magnetic field. This can cause issues for power grids and electronics on Earth, as well as satellites and astronauts in space.
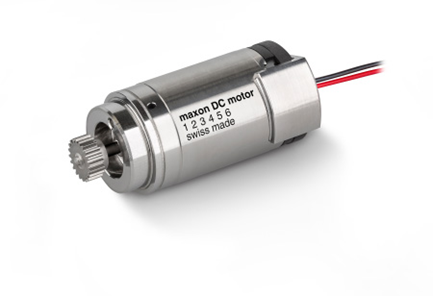
Meanwhile, the motors from Maxon will be powering the opening and closing of a shutter that protects another instrument, a delicate spectrometer-telescope for imaging X-rays whose purpose is to study solar eruptions more closely and may even assist in predicting large-scale eruptions in the future. They are designed to endure five years of challenging conditions.
Magnetometer performs
The first measurements from the magnetometer reached the ground a few days after launch, confirming to the international science teams that it was in good shape following a successful deployment of the spacecraft’s instrument boom.
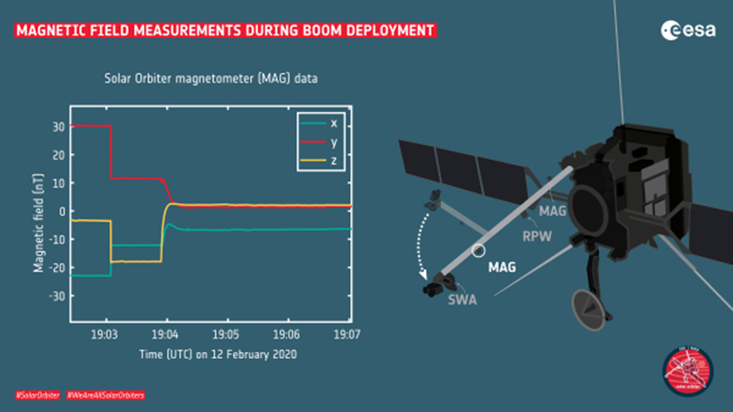
“We measure magnetic fields thousands of times smaller than those we are familiar with on Earth,” says Professor Tim Horbury of the Department of Physics at Imperial College London. He is principal investigator for the magnetometer. “Even currents in electrical wires make magnetic fields far larger than what we need to measure. That’s why our sensors are on a boom, to keep them away from all the electrical activity inside the spacecraft.”
Ground controllers at the European Space Operations Centre in Darmstadt, Germany, switched on the magnetometer’s two sensors, one near the end of the boom and the other close to the spacecraft, about 21 hours after liftoff. The instrument recorded data before, during and after the boom’s deployment, allowing the scientists to understand the influence of the spacecraft on measurements in the space environment.
“The data we received shows how the magnetic field decreases from the vicinity of the spacecraft to where the instruments are actually deployed,” added Horbury. “This is an independent confirmation that the boom actually deployed and that the instruments will, indeed, provide accurate scientific measurements in the future.”
As the titanium/carbon-fibre boom stretched out over an overall 30-minute period almost three days after liftoff, the scientists could observe the level of the magnetic field decrease by about one order of magnitude. While at the beginning they saw mostly the magnetic field of the spacecraft, at the end of the procedure, they got the first glimpse of the significantly weaker magnetic field in the surrounding environment.
“Measuring before, during, and after the boom deployment helps us to identify and characterize signals that are not linked to the solar wind, such as perturbations coming from the spacecraft platform and other instruments,” said Matthieu Kretzschmar, of Laboratoire de Physique et Chimie de l’Environnement et de l’Espace in Orleans, France, lead co-investigator behind another sensor located on the boom, a high frequency magnetometer of the Radio and Plasma Waves instrument, depicted below.
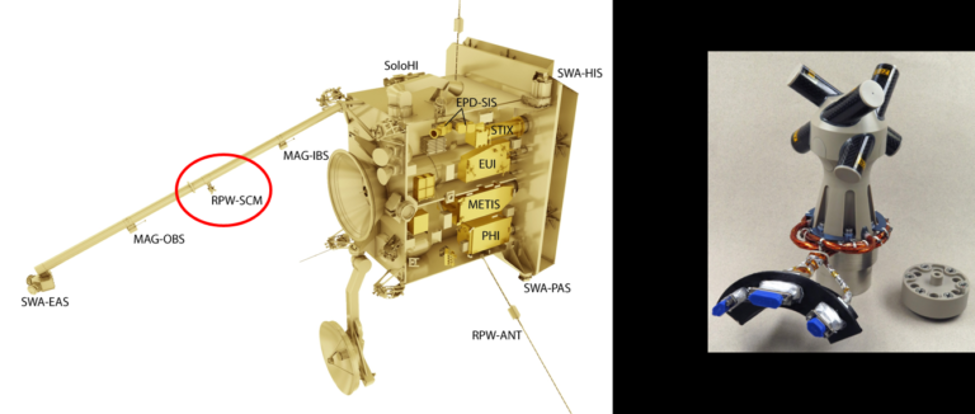
The Espace team is responsible for the manufacturing and operations of a Search-Coil Magnetometer (SCM) that will measure medium and high-frequency magnetic field measurement as part of the radio and plasma wave experiment. The SCM is a three-axis search-coil sensor that will measure the magnetic field from 10Hz to 100kHz, as well as from 10kHz to 500kHz on one of the three axes. Its measurements are crucial for the characterization of the turbulent heating of the solar wind.
“The spacecraft underwent extensive testing on ground to measure its magnetic properties in a special simulation facility, but we couldn’t fully test this aspect until now, in space, because the test equipment usually prevents us from reaching the needed very low level of magnetic field fluctuations,” adds Kretzschmar.
Next, the instruments will have to be calibrated before true science can begin. “Until the end of April, we will be gradually turning on the in-situ instruments and checking whether they are working correctly,” says Yannis Zouganelis, ESA’s deputy project scientist for the Solar Orbiter mission. “By the end of April, we will have a better idea of the performance of the instruments and hopefully start collecting first scientific data in mid-May.”
“The ten instruments onboard our mission will be playing together like instruments in an orchestra,” says ESA Solar Orbiter project scientist Daniel Mueller. “We have just started the rehearsal, and one by one, additional instruments will join. Once we are complete, in a few months’ time, we will be listening to the symphony of the Sun.”
Initial magnetometer data outstanding
“It’s early days, but the data we have so far looks good, commented O’Brien in February. “We are delighted with the measurements and the performance of the instrument – it looks even better than we hoped it would. We’re really excited to be entering the science phase and confident it can carry out its mission well.”
“We spent a long time developing the instrument and it looked good in all the tests we did on the ground, but you never can tell until you get to space,” noted Horbury. “We only have two hours of data so far, but it looks outstanding. The instrument has been well behaved and done all we asked of it. It looks like we will be able to do everything we need, and the instrument will be a key contributor to the science of the mission.”
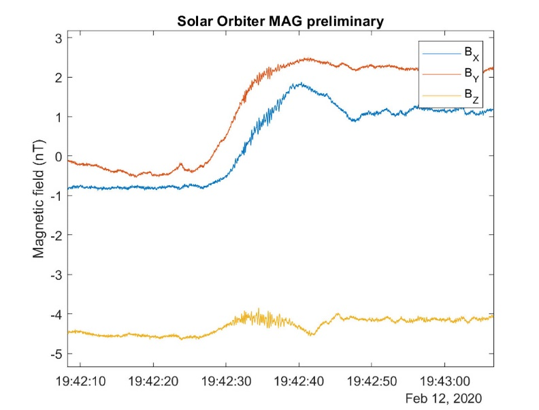
The diagram above shows pristine magnetic field measurements taken in the solar wind by the Imperial magnetometer on Solar Orbiter, more than a million km away from Earth. The plot shows a smooth rotation in the magnetic field, on top of which ride smaller waves. Capturing fine-scale waves such as these, which interact with solar wind particles, is a key target of the Solar Orbiter mission.
Measuring the solar wind
This first data was sent back on a low-bandwidth antenna and arrived instantly. The first science data, however, had to wait until the high-gain antenna was deployed and came down two days later. The magnetometer sits on a long boom at the back of the spacecraft, to keep it away from the other instruments, which could interfere with its readings. The whole spacecraft, which was constructed by Airbus in the UK, and all the separate instruments, had to be very magnetically ‘clean’ – produce no magnetic field of their own – for the magnetometer to work at its best.
We live inside a bubble blown by the Sun, explain the Imperial College scientists. The bubble is made of the solar wind – a stream of charged particles and magnetic field flung out from the Sun’s hot atmosphere. When this tangle of particles and magnetic field reaches us, it interacts with the Earth’s magnetic field. This field protects us, but when the Sun is in a particularly violent mood, extreme solar wind events like flares and coronal mass ejections can interrupt satellites and power grids, affecting our way of life.
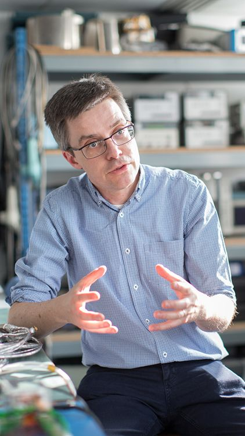
“We’re used to thinking of the Sun as a uniform ball of light, but under the surface it is broiling with complex magnetic fields that can release huge amounts of energy, flinging fast-moving charged particles in our direction, explains,” explains Horbury.
The Earth’s magnetic field can be pictured like a bar magnet. Magnetic field lines come out from one pole, encompass the Earth, and connect back into the other pole. The Sun, however, is writhing with much more tangled magnetic field lines looping in and out across its surface, getting stretched and squeezed and sometimes coming undone, releasing energy and particles. The particles, and with them the magnetic field, sweep out into space, changing and transforming as they go.
But it’s not simply the heat of the Sun the spacecraft and its instruments must contend with, it’s the huge variations of temperature. Out in the vacuum of space, there’s no atmosphere to evenly distribute heat. So, the side of the spacecraft facing the Sun, which is covered by a heat shield, will reach up to 500 degrees C. Just a few meters behind this, out on a boom, the Imperial team’s magnetometer instrument will operate at -140 degrees C.
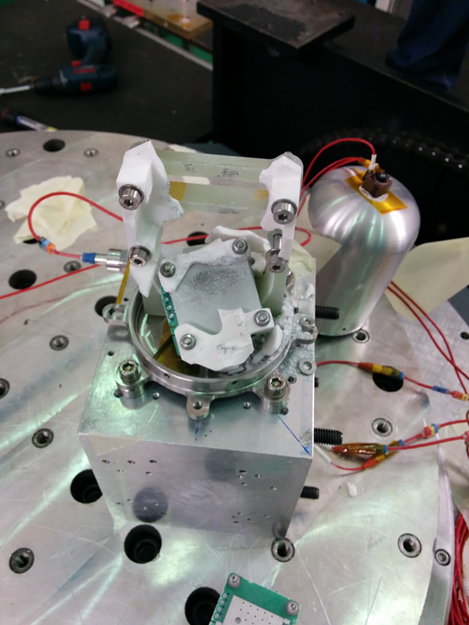
At its surface, the Sun’s magnetic field is around twice that of the Earth’s, but in deep space it is tens of thousands of times smaller, so the magnetometer must be incredibly sensitive. “Our instrument is so sensitive, it could measure the magnetic field of an MRI machine from the other side of London,” notes O’Brien. “This means, however, that we have to work hard to isolate it from the other instruments on the spacecraft. Metal objects and electrical circuits create small magnetic fields, so we have really strict requirements on the rest of the project, right down to the screws and the paint.”
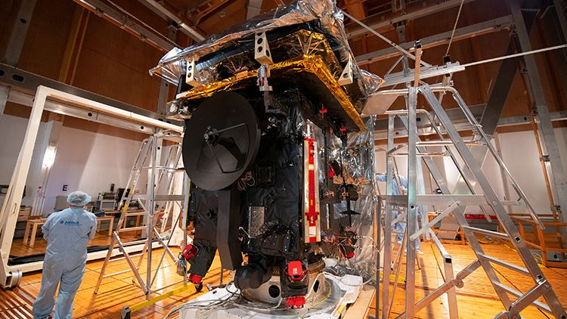
Before launch, as one of the final stages of testing, the spacecraft was placed in a unique facility run by German company IABG in a forest south of Munich to avoid interference with human-generated magnetic fields. The facility consists entirely of non-magnetic materials like wood and contains twelve 15-metre coils, nearly as large as the building, which create a consistent magnetic environment that cancels out the Earth’s own magnetic field, simulating outer space conditions. The magnetometer was then tested while the spacecraft performed some operations that could potentially cause interference, such as extra currents generated by the cameras on board to take images of the Sun.
From the Sun to Mars for Maxon’s micromotors
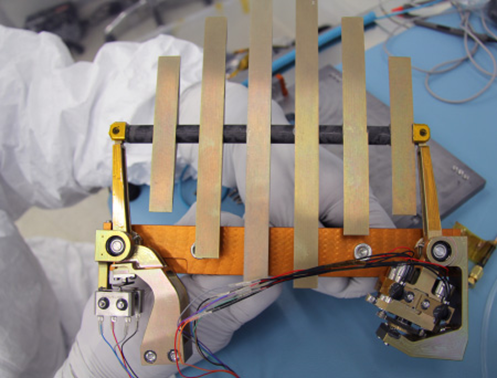
Solar Orbiter will fly within 45 million kilometers of the Sun bringing it closer than Mercury, its nearest planet. At this distance, the side of the probe facing the Sun will be exposed to intense heat, more than 500 degrees C. A heat shield will protect the valuable instruments on board the probe, the shutters provide a view of the Sun only when measurements are being taken.
The drives with Neodymium motors made by Maxon are among the components used in the X-ray telescope. Two specially modified DC motors with diameters of 13 mm move an aluminum attenuator, which slides in front of the telescope’s 30 detectors as required. The micro drives are wired in parallel and can be used together or individually. This ensures that they will run smoothly for the entire five years planned for the mission. The key factors when selecting the drives were their low weight, energy efficiency, and resistance to vibration.
The design is based on micromotors that will soon be headed to Mars. Preparations are underway for the next two major Mars projects which are scheduled to start in the summer of 2020, NASA’s Mars2020 Rover and ESA’s ExoMars Rover. The aim of both missions is to provide new information about the Red Planet including detecting whether life has ever existed there. Mars2020 will also carry a small helicopter to deliver a proof of concept that flights are possible on Mars despite the very thin atmosphere. Maxon drives are used for mission-critical tasks in both projects, including wheel drives, handling of soil samples, and steering the Mars helicopter.
Equipped with their powerful permanent magnets in the core, the centerpiece of the brushed DC motor is its patented ironless rotor. This means cutting-edge technology for compact, powerful drives with low inertia, says Maxon. Due to the low mass moment of inertia, the motors have a very high acceleration. They can be configured in many options.
Solar Orbiter is an ESA-led mission with strong NASA participation. The prime contractor is Airbus Defence & Space in Stevenage, UK. The probe will journey for almost two years before it can start taking measurements. The mission is scheduled to end in 2025.
For more info, see www.esa.int, www.imperial.ac.uk, www.maxongroup.com.