By Brad C. Dodrill, Vice President of Sales & Senior Scientist – Lake Shore Cryotronics
Rare-earth permanent magnet materials are indispensable elements in many electronic devices such as electrical motors, hybrid vehicles, portable communications devices, Aeolian generators used in wind turbines, etc. The magnets have major influence on the size, efficiency, stability and cost of these devices and systems. The development of rare-earth magnets in the 1970s profoundly influenced the application of permanent magnetic materials owing to their large energy product (i.e., BHmax) and increased volume efficiency. The rare-earth magnets that have been most extensively studied and developed are SmCo and NdFeB.
China’s rare-earth export restrictions have led to a resurgence of interest in identifying and exploiting sources of rare-earths outside of China. It also led to the re-opening of Molycorp’s mine in Mountain Pass California, which up until the 1980s was the world’s flagship rare-earth mine. Additionally, there’s renewed interest in developing strong permanent magnet materials that do not rely as heavily on rare-earth constituents, for example nanostructured magnets that would use smaller amounts of rare-earth metals than standard magnets.
Vibrating sample magnetometers (VSM) are the most commonly employed tool for measuring the magnetic properties of permanent magnet materials, because measurements can be performed on solids, powders, single crystals, thin films, nanostructures and liquids. VSMs are also regularly employed because measurements can be performed over a broad range of temperatures (4 K to 1,273 K) that mimic the environmental conditions to which the magnets may actually be exposed. Since measurement of the magnetic properties is exceedingly important in connection with research and development of new magnetic materials, VSMs will prove indispensable in researcher’s efforts to synthesize and develop the next generation of “non-rare-earth” or “rare-earth-light” permanent magnet materials.
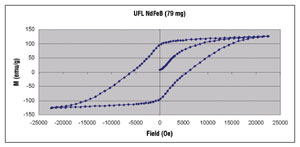
The VSM Technique
If a material is placed within a uniform magnetic field H, a magnetic moment M will be induced in the sample. In a VSM, a sample is placed within suitably placed sensing coils, and is made to undergo sinusoidal motion, i.e., mechanically vibrated. The resulting magnetic flux changes induce a voltage in the sensing coils that is proportional to the magnetic moment of the sample. The magnetic field may be generated by an electromagnet, or a superconducting magnet. Variable temperatures from cryogenic to high temperatures may be achieved using either cryostats or furnace assemblies, respectively.
While a VSM measures magnetic moment m, the quantity of interest is the material’s magnetization M. The magnetization M (in cgs units) can be expressed in terms of the mass (emu/g) or volume magnetization (emu/cc), and is the moment m divided by the sample mass or volume, respectively. A VSM is most commonly used to measure a material’s hysteresis or M(H) loop. In some cases, it is preferred to present the magnetization data in terms of the magnetic induction B, which in cgs units has the unit Gauss (G). The relation between M and B is: B(G) = H + 4Ï€M where M is the volume magnetization (emu/cc).

VSM Measurements of Permanent Magnet Materials
Figure 1 shows the initial magnetization curve, and major M(H) loop for a 79 mg NdFeB powder sample. The most common parameters extracted from the hysteresis loop that are used to characterize the magnetic properties of permanent magnet materials include: the saturation magnetization Ms(the magnetization at maximum applied field), the remanence Mr (the magnetization at zero applied field after applying a saturating field), the coercivity Hc (the field required to demagnetize the sample), and the energy product BHmax which is the magnetic field strength at the point of maximum energy product of a magnetic material. BHmax is obtained from the 2nd quadrant or demagnetization curve of the material (i.e., upper left quadrant in Figure 1) where the curve is recorded starting at remanence Mr and ending at the coercivity Hc. Room temperature 2nd quadrant demagnetization curves for Nd and Sm-based permanent magnet materials are shown in Figure 2.
From this measurement, you would conclude that the Nd-based magnet maintains its properties better when exposed to a higher demagnetizing field. However, this measurement was performed at room temperature. Quite often in real-life applications, the magnets will be exposed to sub-freezing temperatures or to high heat, such as a windmill operating in a northern climate or a starter installed on a vehicle, close to the engine. Thus, VSM variable temperature measurements are often required to fully determine the suitability of a permanent magnet material in the environment within which it will actually be operating.

Figure 3 shows variable temperature measurements for the same Nd and Sm-based magnet materials recorded from -100°C to 600°C. These results show that the Nd and Sm-based magnets have similar properties below and at room temperature. However, once the temperature rises, the Sm-based magnet proves to retain its magnetic properties better than the Nd-based magnet. and is therefore better suited to high temperature applications.
Conclusion
VSMs are well suited to investigate the magnetic properties of permanent magnet materials. The desirable qualities of a permanent magnet material are: high coercivity (Hci), high saturation magnetization (Ms), high remanence (Mr), high energy product BHmax, and for most applications insensitivity to variations in magnetic properties over temperature ranges that the magnet will be subjected to. All of these parameters are easily measured using the VSM technique, and thus the VSM is not only useful for characterizing newly developed materials, but it may also be used for QC/QA process control applications in a manufacturing environment. The VSM will be an important metrology tool for researchers who are developing new permanent magnet materials that do not rely on, or rely less on rare-earth materials.
About the Author
Brad C. Dodrill graduated from The Ohio State University in 1982 with a BSc degree in Physics and a minor in Mathematics. He completed 2 years of graduate studies in Physics and Electrical Engineering and took a position with Lake Shore Cryotronics in 1984 as a Research Scientist. He is presently the Vice President of Sales and a Senior Scientist at Lake Shore. In his technical capacity he is active in applications and product development initiatives in the areas of magnetic and electronic measurements and materials.