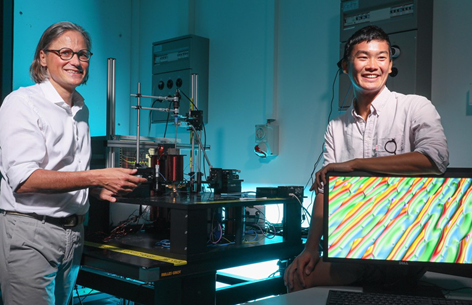
Magnonics researchers at EPFL in Switzerland have shown that electromagnetic waves coupled to precisely engineered structures known as artificial ferromagnetic quasicrystals allow for more efficient information transmission and processing at the nanoscale. Their research also represents the first practical demonstration of Conway worms, a theoretical concept for the description of quasicrystals.
Magnonics is an emerging field of magnetism that is similar to, but slightly different from, spintronics. While spintronics makes use of an electron’s electric charge and spin moment properties to encode data, magnonics makes use of the amplitude and phase of spin-wave signals in magnetic materials.
High-frequency electromagnetic waves are used to transmit and process information in microelectronic devices such as smartphones. It’s already appreciated that these waves can be compressed using magnetic oscillations known as spin waves or magnons. This compression could pave the way for the design of nanoscale, multifunctional microwave devices with a considerably reduced footprint. But first, scientists need to gain a better understanding of spin waves, or precisely how magnons behave and propagate in different structures.
In a study conducted by doctoral assistant Sho Watanabe, shown at right, postdoctoral researcher Dr. Vinayak Bhat, left, and other team members, the scientists from EPFL’s Laboratory of Nanoscale Magnetic Materials and Magnonics examined how electromagnetic waves propagate, and how they could be manipulated, in precisely engineered nanostructures known as artificial ferromagnetic quasicrystals. The quasicrystals have a unique property: their structure is aperiodic, meaning that their constituent atoms or tailor-made elements do not follow a regular, repeating pattern but are still arranged deterministically. Although this characteristic makes materials especially useful for the design of everyday and high-tech devices, it remains poorly understood.
The LMGN team found that, under controlled conditions, a single electromagnetic wave coupled to an artificial quasicrystal splits into several spin waves, which then propagate within the structure. Each of these spin waves represents a different phase of the original electromagnetic wave, carrying different information.
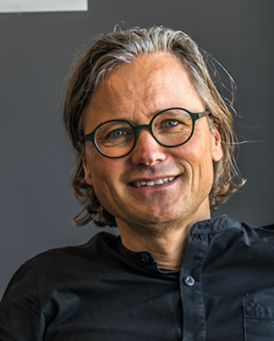
“It’s a very interesting discovery, because existing information-transmission methods follow the same principle,” says Dirk Grundler, an associate professor at EPFL’s School of Engineering. “Except you need an extra device, a multiplexer, to split the input signal because, unlike in our study, it doesn’t divide on its own.”
Grundler also explains that, in conventional systems, the information contained in each wave can only be read at different frequencies – another inconvenience that the EPFL team overcame in their study. “In our two-dimensional quasicrystals, all the waves can be read at the same frequency,” he adds. The findings have been published in the journal, Advanced Functional Materials.
The researchers also observed that, rather than propagating randomly, the waves often moved like so-called Conway worms, named after a well-known mathematician John Horton Conway who also developed a model to describe the behavior and feeding patterns of prehistoric worms. Conway discovered that, within two-dimensional quasicrystals, constituent elements arrange like meandering worms following a Fibonacci sequence. Thereby they form selected one-dimensional quasicrystals. “Our study represents the first practical demonstration of this theoretical concept, proving that the sequences induce interesting functional properties of waves in a quasicrystal,” says Grundler. For more info, see www.epfl.ch.