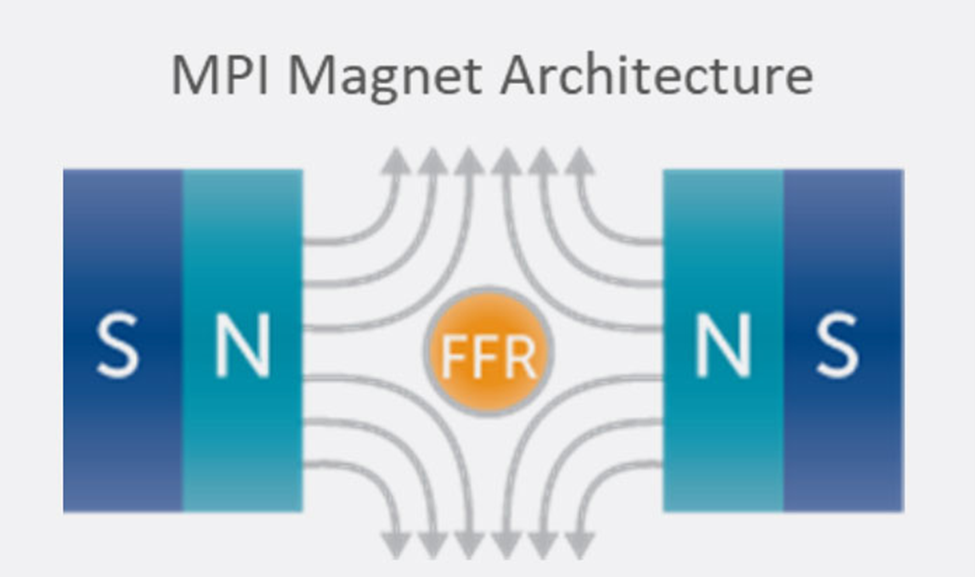
Magnetic Insight, a pioneering developer of magnetic particle imaging technology, has made significant advancements recently as it prepares to make the big jump from pre-clinical to clinical use. The company reports several developments including the appointment of Chris Raanes as the new CEO and $3 million in grant funding. The funds include a joint grant with The University of California at Berkeley to dramatically improve the spatial resolution of MPI, and a National Institute of Health grant to drive the sensitivity of MPI to the physics limits.
MPI is an innovative tracer imaging technology that offers the sensitivity of nuclear medicine without using radioactive tracers. In contrast to traditional methods, it offers enhanced image quality, simple logistics without time-critical deliveries, and no radiation exposure, enabling new applications and improving patient and doctor experience while reducing overall healthcare costs.
The CEO appointment primes the company, based in Alameda, California, for transition from preclinical to clinical application. Raanes has over twenty years of experience in the medical device sector, having led companies through pivotal stages of growth and commercialization. Previously, he served as Spire Health’s CEO where he supported the commercialization of a wearable sensor for respiratory patients. As CEO of ViewRay, he brought the company public and led the commercial introduction of an MRI-guided radiation therapy system. He holds a B.S. and an M.S. in Electrical Engineering from the Massachusetts Institute of Technology.
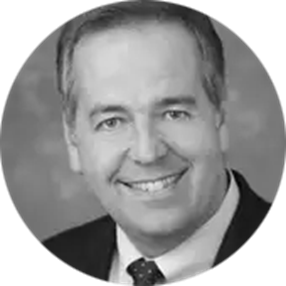
“I’m honored to join the Magnetic Insight team, especially at such a pivotal time for groundbreaking research and advancements in the MPI space,” said Raanes. “This technology has the potential to reimagine medical imaging, expanding the field’s capabilities and providing an alternative to using radiation in many critical applications.”
The NIH funding is for a multi-million-dollar Phase II Small Business Innovative Research grant to develop the engineering underpinning their clinical MPI imaging platform development. The project addresses the absence of a clinical MPI scanner by building the low-noise electronics necessary for a general-purpose clinical MPI scanner, leveraging innovations in transmit-receive subsystem design and implementation.
The grant award augments funding from a separately announced collaborative NIH grant with the University of California Berkeley to dramatically improve MPI spatial resolution and accelerate imaging applications for CAR-T cell therapies, stroke, gastrointestinal bleeds and pulmonary embolisms, and an ongoing collaborative NIH Phase II SBIR grant with Johns Hopkins to integrate magnetic fluid hyperthermia into Magnetic Insight’s clinical imager under development to expand the application of MPI beyond cancer diagnosis to include cancer treatment.
Imaging without radiation
Magnetic particle imaging is viewed as a potentially groundbreaking non-radioactive alternative that can potentially replace over half of nuclear medicine scans and enable entirely new classes of imaging applications. It addresses not only supply vulnerabilities but also the imaging experience for both healthcare professionals and patients. Having already provided MPI systems for small animal imaging to universities globally, Magnetic Insight now seeks to transition the technology to the clinic. Fifteen Magnetic Insight MPI scanners have been installed worldwide at leading universities, including Stanford University, Johns Hopkins University, and the Karolinska Institute.
How does MPI work?
As explained by Magnetic Insight, an MPI imager directly detects a tracer known as a super-paramagnetic iron oxide particle or SPIO. The technology detects only the SPIOs and does not see tissue. These tracers can be modified to suit the application desired. For example, suppose tracers selectively label immunotherapy cells such as CAR T-cells. Distribution of those cells after patient administration can be imaged. This article highlights some of the company’s explanation; a whitepaper on their website provides more detail.
Key to the scanner operation is the strong magnetic field gradient produced inside the imaging volume by the main magnet. Inside this magnetic field gradient, a small region with low magnetic field strength exists, known as the field-free region or FFR. Outside of the FFR, the magnetic field is strong.
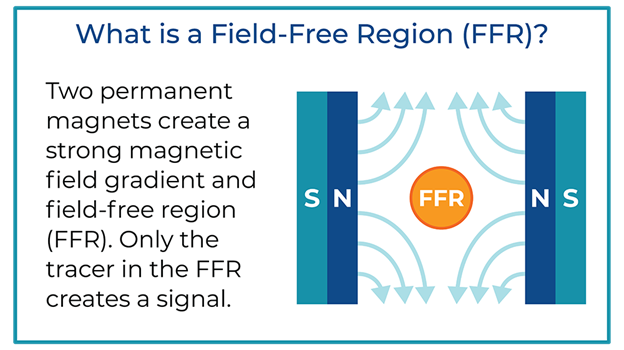
The technique is fundamentally different from magnetic resonance imaging (MRI) despite apparent similarities in acronyms and the use of magnets. MRI detects the resonance of nuclear spins and is generally used for anatomical imaging. MPI detects magnetization in nanoparticles and is used mainly for cellular imaging. The two technologies also use different main magnet architectures.
In MPI, two strong magnets pointing at each other produce a magnetic field gradient with an FFR at the center. The FFR is then rapidly moved across the sample to produce an image using strong gradients (T/m) and weak field strengths (mT). The imaging system’s resolution, sensitivity, and contrast are driven by the interaction between the imager’s main field gradient and the SPIO tracer’s magnetic properties. Additionally, the ability of a tracer to visualize an application, such as looking at the immune system, is driven by the nanoparticle’s behavior in tissue.
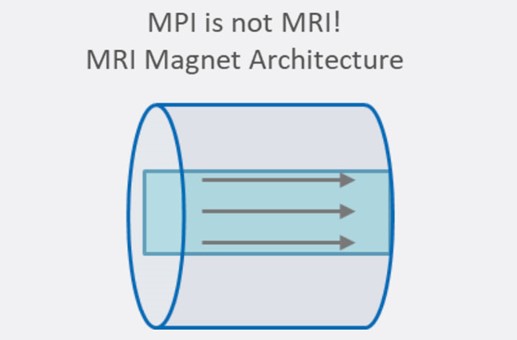
MPI tracer nanoparticles
MPI detects magnetic tracers, many of which are biocompatible and biodegrade in vivo, making them an ideal material for clinical imaging. SPIO nanoparticles are a type of magnetic tracer with a long history in medical imaging and with FDA and EU-approved formulations. In contrast to radioactive tracers, MPI tracers are manufactured using standard chemistry equipment. For preclinical use, Magnetic Insight offers the nanoparticles in two formats, Vivotrax and Vivotrax Plus.
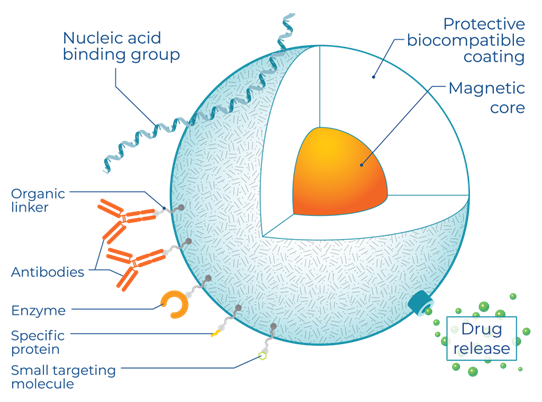
Magnetic Core Properties
Unlike other imaging approaches, MPI image quality is dependent on the magnetic properties of its tracer. The core diameter, magnetic material, oxidation state, and crystallinity are crucial. This means that advances in the formulation of SPIOs can drive substantial improvements in MPI sensitivity and resolution, independent of any changes in the MPI imaging hardware. This has resulted in significant nanoparticle development efforts by research groups worldwide.
Nanoparticle Coatings
The ability to use MPI to track the progress of cellular therapy is tied to the development of SPIOs that are stable in cells and remain active for imaging over days to weeks, explains Magnetic Insight. For example, this can require coatings that prevent cells from degrading SPIOs too quickly while still allowing for robust cell labeling. Coatings such as polyethylene glycol prevent nanoparticle aggregation due to magnetic interactions and enhance colloidal stability.
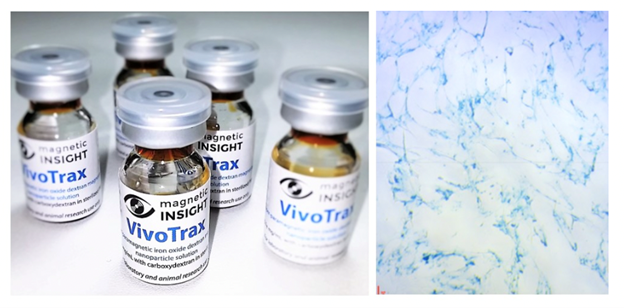
Momentum Imager specialized scanning equipment
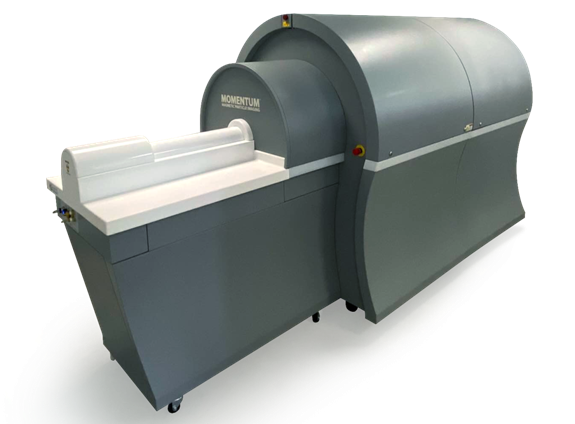
Magnetic Insight’s Momentum CT is a self-shielded pre-clinical MPI imager with integrated X-ray/CT. The imaging system utilizes a high-field gradient main magnet and proprietary x-space image reconstruction to produce high-resolution images. The static magnetic field gradient, known as a “selection” field, balances the competing requirements of image resolution, signal strength, and scanning speed. A pair of water-cooled electromagnets and a laminated iron field generate a 6 tesla/meter selection field gradient, providing sufficient field strength to produce a small FFR for millimeter-scale image resolution. For more info, see www.magneticinsight.com.