A Case Study by Cathleen Lambertson • Comsol, Inc.
Since 1995, Advanced Magnet Lab (AML) has provided technology for the design and manufacture of advanced coils, magnets and magnet systems for the energy, medical, defense and research industries. The company’s 3D coil-design software and automated construction processes enable rapid deployment of complex state-of-the-art magnets.Recently, AML developed a new magnet topology, Direct Double- Helix (DDH) magnets, which allow for a significant increase in power density, performance in field generation and field quality. The unique characteristics of the DDH magnets, part of the Double- Helix (DH) magnet family invented by AML’s founder, Dr. Rainer Meinke, could lead to more affordable systems and potentially portable devices. The magnets have a large number of applications including high-speed generators and charged particle beam optics. AML’s customers include the Department of Energy, NASA, the European Organization for Nuclear Research (CERN), Center for Advanced Power Systems (CAPS), National High Magnetic Field Laboratory (NHMFL) and GE Medical Systems.

The Technology
Unlike conventional magnets based on saddle or racetrack coil configurations, AML’s processes and designs enable magnets of any multipole fields with unmatched field homogeneity. Specifically, the DH and DDH magnets are composed of modulated tilted helices (Figure 1) that produce magnetic fields with very pure multipole content. However, while DH magnets are based on very accurate positioning of wire in machined grooves, DDH technology enables the design and manufacturing of magnet coils in one step without conventional conductor and winding processes.

“DDH magnets are created in-situ and conducting paths are machined directly out of a resistive object such as a conductive cylinder. The machined grooves serve as electrical insulation and follow a mathematical equation leading to non-uniform cross section of the conductive paths,” said Dr. Philippe Masson, a Senior Research Scientist at AML. This results in a reduction of the total electrical resistance of a DDH magnet, meaning more current can be flown into the conductor; hence, smaller magnets are produced. “Another great benefit is that each layer of a DDH magnet presents a large surface allowing for improved cooling. With a simple watercooling system, small DDH coils can carry an excess of 200 A/mm2 of peak current density in steady state,” said Masson.
Optimized Magnet Design

According to Dr. Masson, when DDH was invented, the advantages were clear in concept: outstanding performances were obtained experimentally and applied to commercial products. However, in order to really understand the physics behind the measurements, a detailed numerical analysis was required.
COMSOL Multiphysics was used to visualize the current distribution in the magnet (Figure 2) and to simulate the heat transfer in steady state operation through the coupling of the Conductive Media DC and General Heat Transfer physics interfaces. The main question is, what is the limit of the technology?
“Magnet design is a multiphysics process dealing with electromagnetism, thermal analysis, structural analysis, and fluid dynamics. The software allowed for all those aspects of the design to be simulated, and moreover, to be coupled together, making it the ideal tool for a magnet company,” said Dr. Masson.
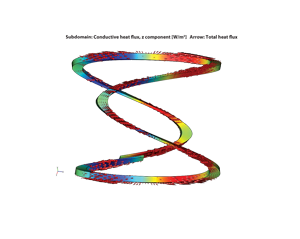
Since the resistance of the DDH coils strongly depends on the current density distribution, the first question Dr. Masson and his team needed to answer was how the current actually distributed in the conductor and how to accurately predict the resistance. Secondly, the team needed to figure out how the non-uniform distribution of current density in the conductor affected the field homogeneity. Finally, they worked on how the heat propagates in the coil and to the coolant. “All these questions were answered and the results are used to help push the limits of the technology and improve the coil design,” said Dr. Masson. “Using COMSOL Multiphysics allows for the design to be optimized numerically before anything is actually fabricated. All the trial and error can be performed through simulation, thus saving time and money.”
For more information on this case study or about Comsol Multiphysics, please visit www.comsol.com. For more information on the Direct Double Helix and Advanced Magnet Lab, please visit www.magnetlab.com.
Published in the Spring 2012 Issue