By Rocco Holzhey | Innovent e.V.
Permanent magnets are widely used for sensor and motor applications. Besides these, magnetic fields are also used for applications such as storage of information and fraud protection on documents. With the number of critical applications continuing to rise, developers and producers are increasingly forced to meet safety requirements, as well as increase the technology’s overall efficiency. In order to ensure the high quality that the end user demands, comprehensive quality control is necessary.
Depending on the application, it is possible and often necessary to measure magnets in the far and in the near field.
In this blog I will describe a new innovative measuring technique to characterize magnets in the far field with a stray field measurement and a dipole approximation.
For the so called far field measurements, there is a large distance between the source object and the measurement position. A large distance in this sense means a distance from at least five times the biggest dimension of the object. In this distance the magnetic stray field of a permanent magnet is a dipole. With this assumption it is possible to characterize a permanent magnet based on the measurement with an array magnetoresistiv sensor elements. Similar to helmholtz coil fluxmeter combinations this method provide the magnetization error (angle of magnetization) and the open remanence of the magnet in a very easy, fast and precise way. Compared with helmholtz coils a build up of an automated inline measuring system is possible. With such a fast measurement the magnets for motor application can be measured, sorted and screened very fast. It seems with such a measuring and sorting a reduction of the magnet material in a motor and a prevention of cogging effects are possible.
Theoretical Background “Magnetic Monitoring”
Within the magnetic monitoring technique, an array of magnetic field-sensitive sensors is used to track small dipolar permanent magnets. At the same time the characteristics of the specimen itself can be measured. For the localization algorithm, the position and orientation of the magnetic dipole constitute five independent parameters. If the magnitude of the magnetic dipole moment of the specimen is not known or is subject to variation during the measurement, this quantity constitutes a sixth parameter, which must be determined. Mathematically, it would be sufficient to use six magnetic field sensors in order to determine the six unknown parameters. In practice, more sensors are used for three reasons: Additional information is needed to determine and compensate for disturbing fields, additional magnetic sensors can provide homogeneous localization sensitivity within the desired measurement volume almost independent of the orientation of the specimen, and statistical deviations of the field values measured by the individual sensors, e.g. due to noise, are strongly reduced.
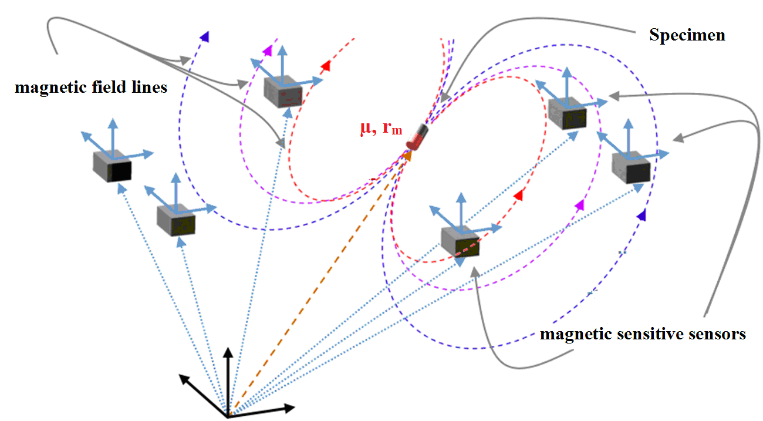
Figure 1 shows the principle of the magnetic monitoring technique. In a sufficiently large distance from a permanent magnet with magnetisation M (vector: strength and direction) and volume V, only the contribution of its magnetic dipole moment µ = M · V to the total magnetic field can be measured.
The magnetic flux density generated by a magnetic dipole is
with r and r = |r|, being position vector and distance with respect to the position of the magnetic dipole. Since more sensors than unknown dipole parameters are used, the localization is performed by a least squares optimum search algorithm. The dipole moment µ and position rm of a dipole are determined such, that the field generated by the dipole fits best the fields measured at the sensor positions rs. A measure for the quality of the localization is determined by the quality function Q:
Suppression of Disturbing Fields
The field of the magnetic marker is overlaid with steady and time varying fields of different shape. Steady fields result from the earth magnetic field and its deformations by iron structures (e.g. concrete reinforcement). If such structures move (cars, elevators, hospital beds etc.), the respective fields change in time. Slowly varying fields may also origin from tramways, which work with DC current and poses a large coil between rails and overhead wire, with changing length and current. The homogeneity of the disturbing fields depends on the distance of the field source; near sources produce more inhomogeneous fields.
Distortions due to steady fields can be suppressed by taking a baseline prior to the measurement. Homogeneous time varying fields are handled by a homogeneous field suppression algorithm. If size and shape of the sensor field provide enough information, also inhomogeneous time varying field sources may be separated by adaptation of the multipole expansion method.
Magnet Characterization Systems Based on “Magnetic Monitoring”
Based on the described algorithm in combination with a sensitive array of magnetic field sensors it is possible to build up sensitive measuring devices to characterize permanent magnets. Figure 2. shows a measurement system (m-axis, / www.matesy.com), which is developed to measure the magnitude and orientation of the magnetic dipole moment very precisely in a QM environment. This system is developed to probe single magnets with a hand feeding of the specimen on a human working place. In principle the measuring frequency is high enough to use this device in an automated 100 percent inline measurement process.

Based on the m-axis technology a special inline system was developed and optimized for a 100 percent inline measurement of the specimen. The system consists of six measuring boards with three highly sensitive AMR sensors each. The flux densities that can be measured with AMR sensors range from 20 nT up to 400 µT. The data of the AMR sensors is collected simultaneously for all 18 AMR sensors. The overall measuring frequency is 100 Hz. A connection to a superordinate system is possible via a standard USB or Ethernet.
About the Author
Rocco Holzhey graduated in Environmental Technologies at University of Applied Sciences Jena in 2005 and in a Master Thesis in Sientific Instrumentation in 2008.
Rocco joined INNOVENT e.V. Technology Development Jena, which is a well known non-profit, industrial research association founded in 1994 in Jena. At INNOVENT currently 150 employees works in 5 research departments in the fields of Magnetic and Optical Systems, Surface Engineering, Biomaterials and Analytics. INNOVENT is one of the largest private research institutes in Germany.
Since 2006 he works on the field of magnetic materials, simulations, magnetic measuring techniques and systems.
Since 2014 he is Head of Department MOS (Magnetic an Optical Systems) at INNOVENT e.V.
Rocco can be reached at rh2@innovent-jena.de / and Phone: +49 (0) 3641/282515