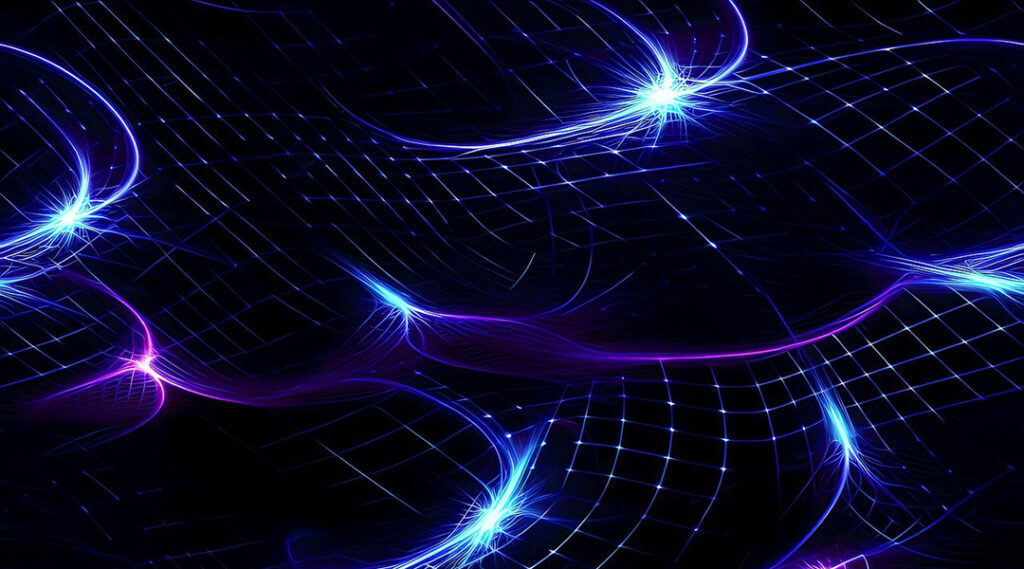
By Andrey Feldman
Magnetism plays a pivotal role in how a computer functions — how atoms and electrons react to magnetic fields enables the reading, writing, and manipulation of data in electronic devices.
Researchers have demonstrated for the first time how exposing a typically non-magnetic material to high-frequency laser radiation can induce magnetism at room temperature.
This breakthrough holds promise for revolutionizing electronics, especially those based on quantum technology, which typically require temperatures around absolute zero (-273 degrees Celsius) to operate.
Turning insulators into magnets
In their study, the researchers subjected a material known as strontium titanate to light from a high frequency laser, “stirring up” the atoms and mobilizing them to generate electric currents within the material, making it magnetic.
“The innovation in this method lies in the concept of letting light move atoms and electrons in this material in circular motion, so to generate currents that make it as magnetic as a refrigerator magnet,” Stefano Bonetti, a physicist at Stockholm University and at the Ca’ Foscari University of Venice and lead author of the study, explains in a press release.
The possibility of making non-magnetic materials magnetic had been previously predicted. However, this is the first time this theory has been proven experimentally.
“We have been able to do so by developing a new light source in the far-infrared with a polarization, which has a ‘corkscrew’ shape,” said Bonetti. “This is the first time we have been able to induce and clearly see how the material becomes magnetic at room temperature in an experiment. Furthermore, our approach allows us to make magnetic materials out of many insulators, when magnets are typically made of metals.”
To measure the degree of magnetization induced using their laser technique, the physicists relied on a well-known effect in which light reflects differently off a material depending how magnetic it is. Their measurements showed that the material had indeed become magnetic, but the magnitude of induced magnetization turned out to be about four orders of magnitude greater than expected based on known theoretical methods for calculating this quantity.
The team attributed this discrepancy to oversimplifications they made in their calculations and that a detailed account of the effects associated with the interaction of electrons with the crystal lattice of the material could resolve the contradictions that arose between experiment and theory.
Where will light-induced magnetization find application?
The scientists report they were able to maintain magnetism in the material for about one trillionth of a second, which is not yet long enough for use in applications such as computer memory, but is a significant starting point in which theory has been brought to reality, with important potential technological applications.
For one, strontium titanate could just be the beginning, serving as the basis for exploring more complex materials that could potentially retain magnetism for longer periods of time. There is also the potential to use this light-based approach to induce magnetization in other non-strontium titanate materials, some of which will hopefully be able to remain magnetic for much longer, opening the door to use in computing devices.
“This opens up for ultra-fast magnetic switches that can be used for faster information transfer and considerably better data storage, and for computers that are significantly faster and more energy-efficient,” said Alexander Balatsky, professor of physics at NORDITA and an author of the study in a press release.
Reference: M. Basini et al., Terahertz electric-field driven dynamical multiferroicity in SrTiO3, Nature (2024). DOI: 10.1038/s41586-024-07175-9
Feature image credit: SuttleMedia on Pixabay