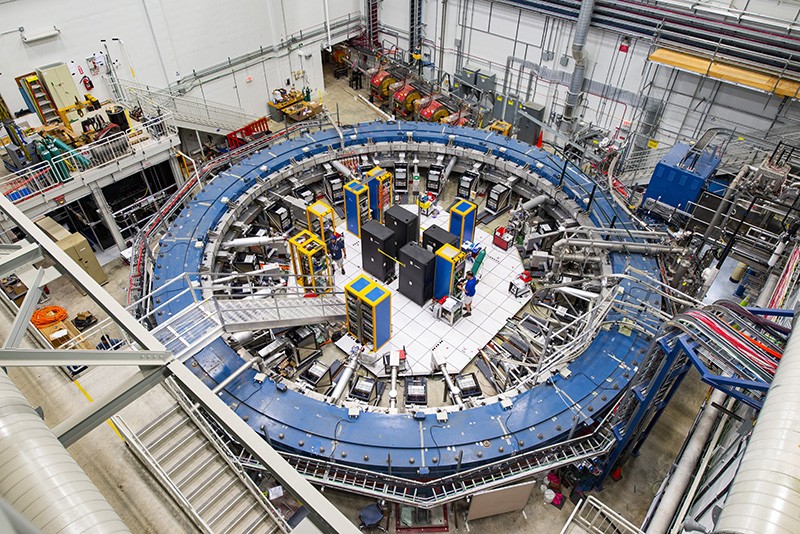
Muons keep on misbehaving. An experiment in the United States has confirmed an earlier finding that the particles — massive, unstable cousins of the electron — are more magnetic than researchers originally expected. If the results hold up, they could ultimately force major changes in theoretical physics and reveal the existence of completely new fundamental particles.
The Muon g − 2 collaboration at the Fermi National Accelerator Laboratory (Fermilab) outside Chicago, Illinois, reported the latest measurements in a webcast on 7 April, and published them in Physical Review Letters. The results are “extremely encouraging” for those hoping to discover other particles, says Susan Gardner, a physicist at the University of Kentucky in Lexington.
Muon g − 2 (pronounced ‘g minus 2’) first hinted that something was amiss with the muon in 2001, when the experiment was running at the Brookhaven National Laboratory in Upton, New York. Physicists measured the strength of the particle’s magnetic moment, a property that makes it act like a tiny bar magnet. The standard model of particle physics says that, in the appropriate units, the muon’s magnetic moment should be a number very close, but not equal, to 2. The Brookhaven experiment measured that tiny difference, known as g – 2, but found it to be slightly bigger than theorists had predicted.
The magnetic moment of elementary particles is influenced by ‘virtual’ versions of known elementary particles that continually pop out of the vacuum only to disappear a fraction of a second later. Physicists perform detailed and lengthy calculations of the contributions from all known particles, so if the experimental results differ significantly from the predicted value of g − 2, they reason that previously unknown types of particle must be lurking in the vacuum. The original Muon g − 2 experiment gave many physicists hope that new particles would soon be discovered.
Secret frequency
To verify the Brookhaven results, researchers rebuilt the experiment — which keeps muons running in circles around a superconducting ring magnet 15 metres in diameter — at Fermilab. They began collecting data in 2018, and have now presented the results from the first year of operations.
To avoid biasing its data analysis, the collaboration had blinded itself to a crucial parameter that is needed to calculate the g − 2 constant — the exact frequency of a digital clock in their instrumentation. Two Fermilab physicists who are not collaboration members were entrusted with the missing bit of information. As a result, the team was able to conduct a lengthy study, but could initially plot its findings only on a graph in which the axes had slightly uncertain scales.
Then at a 25 February teleconference that included most of the 200-plus team members, two leading members of the experiment opened an envelope that contained the secret clock frequency. When they plugged the number into their computers, it revealed the true value of their g − 2 measurement. It was immediately obvious to the team that the result was consistent with the one recorded at Brookhaven more than 20 years ago.
“The agreement is excellent,” says Lee Roberts at Boston University in Massachusetts, one of the original Muon g − 2 team members. “People were clapping and jumping up and down — as much as you can do that on Zoom.” The joyful reactions were obvious, even though “a lot of us were muted”, adds Brynn MacCoy, a physicist at the University of Washington in Seattle. The result vindicates the claim of the original experiment, Roberts says.
Other physicists agree. The latest announcement gives “a nice, clear answer” to the riddle posed by the earlier results, says theoretical physicist Gino Isidori at the University of Zurich in Switzerland. “The experiment was correct.”
But although the gap between the theoretical and experimental results has grown in statistical significance, it is still not an unambiguous proof of the existence of new particles. “Those who were sceptical will probably stay sceptical,” Isidori says. “At this point, the ball is in the theorists’ court,” he adds.
Quark calculations
The most widely accepted prediction for the muon’s magnetic moment is a number that the theoretical community published last year in a ‘consensus’ paper. But another study published on 7 April, this time in Nature, suggests that the gap between theory and experiment might not be as large as thought.
The hardest part to calculate is the contribution of quarks, the basic constituents of protons and neutrons, which is why physicists have conventionally supplemented their calculations with data from collider experiments.
In the Nature study, Zoltan Fodor at Pennsylvania State University in University Park and his collaborators recalculated the quark contributions from scratch with a simulation technique called lattice quantum chromodynamics (lattice QCD). The technique had not previously been used in g − 2 predictions because it was not mature enough to give high-precision results. Fodor and his team managed to improve the precision, and found g − 2 to be both larger than the consensus value and much closer to the experimental measurement. Other lattice QCD teams are working to match that precision so that the technique can be used in calculations for the consensus value, says Aida El-Khadra, a theoretical physicist at the University of Illinois at Urbana–Champaign. “The other collaborations are also working on reducing their errors, which requires significant computational resources,” she says.
Updating the physics
The Muon g − 2 team is now busy analysing some of the more recent data, as well as collecting more. The researchers ultimately expect the precision of their measurement to improve fourfold. If the discrepancy does turn out to be real, then the standard model will have to be updated to include new particles. One problem is that since 2001, many possible candidate particles that could have inflated the muon’s magnetic moment have been ruled out in other experiments, mostly by the Large Hadron Collider outside Geneva, Switzerland.
Many theories that could explain the Muon g − 2 results remain, but researchers see them as contrived. “To me there is not a single explanation which stands out as being far more elegant or compelling than any other one,” says Dominik Stöckinger, a theoretical physicist at the Dresden University of Technology in Germany who is a member of Muon g − 2.
Since it was first put together in the 1970s, the standard model has passed all tests and has survived almost unchanged. But physicists are convinced that it must be incomplete, and some hope that muons will reveal its first failure. “If we confirm a difference with the standard model, that’s what people have been searching for for 50 years,” says Roberts.
Photo: The storage-ring magnet used for the g − 2 experiment at Fermilab. Credit: Reidar Hahn/Fermilab