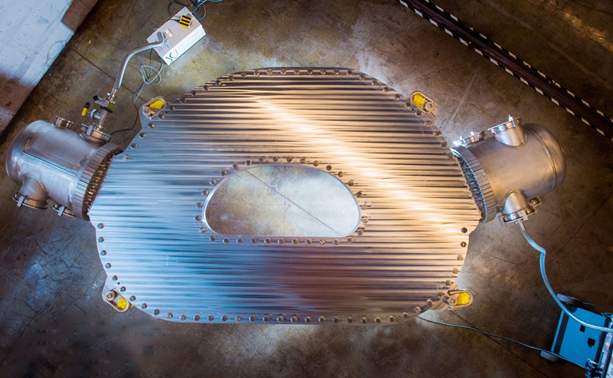
With more than $1.8 billion in blue-ribbon investor funding and successful demonstrations of the practicality of a record-powerful magnet, Commonwealth Fusion Systems, a spinoff from Massachusetts Institute of Technology, is trailblazing a path toward commercializing fusion energy. It plans to reach the long-coveted destination in the early 2030s. Two big steps were accomplished recently – one involves money, the other technology using high-temperature superconducting tape.
In November, it closed on more than $1.8 billion in Series B funding from an array of high-profile energy investors, providing capital to construct, commission, and operate SPARC, planned to become the world’s first commercially relevant net energy fusion machine. In addition, it will enable the company to begin work on ARC, planned to become the first commercial fusion power plant. Among the blue-ribbon participants are Bill Gates, Google, Marc Benioff’s TIME Ventures, Khosla Ventures, Soros Fund Management and several large energy investment funds. CFS has raised more than $2 billion in funding since it was founded in 2018.
“The world is ready to make big investments in commercial fusion as a key part of the global energy transition. This diverse group of investors includes a spectrum of capital from energy and technology companies to venture capitalists, hedge funds, and university endowments that believe in fusion as a large-scale solution to decarbonize the planet,” said CEO Bob Mumgaard.
Only two months earlier, CFS and the Plasma Science and Fusion Center (PSFC) at Massachusetts Institute of Technology announced the successful test of the world’s strongest high temperature superconducting (HTS) magnet, the key technology for the device that they expect to unlock the path to clean commercial fusion energy. Conducted at the MIT center, the test proved that the magnet built at scale can reach a sustained magnetic field of more than 20 tesla, making it the strongest magnet in the world and powerful enough to enable CFS’s compact tokamak device, called SPARC, to achieve net energy from fusion, a historic first.
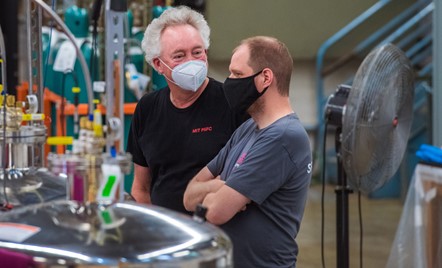
“This record-breaking magnet is the culmination of the last three years of work and will give the world a clear path to fusion power for the first time,” said Mumgaard. “The world needs a fundamentally new technology that will support efforts to decarbonize on a timeline that can mitigate climate change. This test of our magnet proves we have that technology, and we’re on our way to producing clean, limitless energy for the entire world.”
New commercially available high temperature superconducting tape was used to build the magnets that will enable significantly stronger magnetic fields. While existing tokamaks rely on device scale to attempt net energy, HTS magnets enable a high-field approach that will enable CFS to reach net energy from fusion with a device that is substantially smaller, lower cost, and on a faster timeline. “This groundbreaking magnet opens a widely identified transformational and accelerated opportunity for advancing fusion science and commercial fusion energy,” said Whyte, who is also a co-founder of CFS.
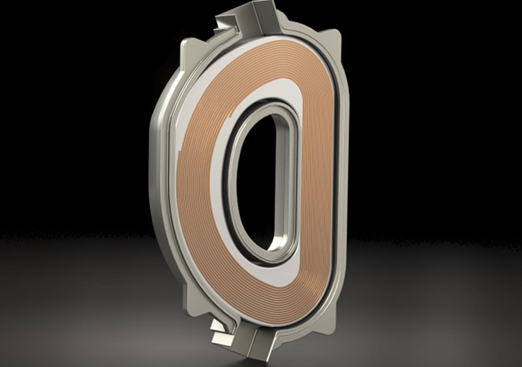
Tokamaks are donut-shaped devices that use magnets to control and insulate a plasma in which fusion occurs. While no fusion device has yet to achieve net energy from fusion, tokamaks have come the closest with more than 160 tokamaks built and successfully operated around the world. The new HTS magnets can enable significantly stronger magnetic fields and as a result significantly smaller tokamaks.
Superconducting magnets are key
Fusion, the process that powers the sun and stars, involves light elements, such as hydrogen, smashing together to form heavier elements such as helium and releasing prodigious amounts of energy in the process. This process produces net energy only at extreme temperatures of hundreds of millions of degrees Celsius, too hot for any solid material to withstand. To get around that, fusion researchers use magnetic fields to hold in place the hot plasma which can be likened to a gaseous soup of subatomic particles, keeping it from contacting any part of the donut-shaped chamber.
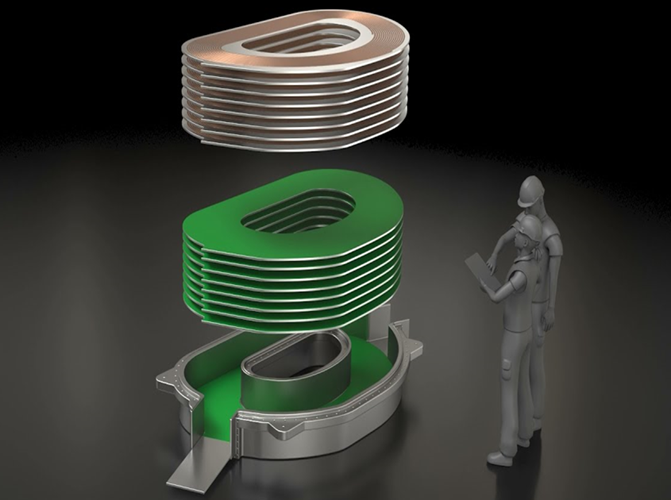
Developing the new magnet is seen as the greatest technological hurdle to making that happen; its successful operation now opens the door to demonstrating fusion in a lab, which has been pursued for decades with limited progress. “The challenges of making fusion happen are both technical and scientific,” says Whyte, but once the technology is proven, “it’s an inexhaustible, carbon-free source of energy that you can deploy anywhere and at any time. It’s really a fundamentally new energy source.” The recent demonstration represents a major milestone, addressing the biggest questions remaining about the feasibility of the SPARC design. “It’s really a watershed moment, I believe, in fusion science and technology.”
Most tokamaks use conventional electromagnets made of copper, but the latest and largest version, under construction in France in a multinational scientific collaboration called ITER, uses low-temperature superconductors. For that project, General Atomics of San Diego, California completed last year the first module of the central solenoid, capable of reaching a magnetic field strength of 13 tesla, at the time considered the world’s most powerful magnet.
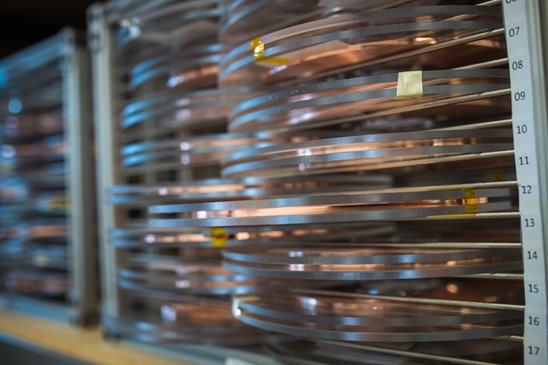
The major innovation in the MIT-CFS fusion design is the use of high-temperature superconductors which enable a much stronger magnetic field in a smaller space. This design was made possible by a new kind of superconducting material that became commercially available a few years ago. Until now, the only way to achieve the colossally powerful magnetic fields needed to create a magnetic “bottle” capable of containing plasma heated up to hundreds of millions of degrees was to make them larger and larger. But the new HTS material, made in the form of a flat, ribbon-like tape, makes it possible to achieve a higher magnetic field in a smaller device, equaling the performance that would be achieved in an apparatus many times larger using conventional low-temperature superconducting magnets.
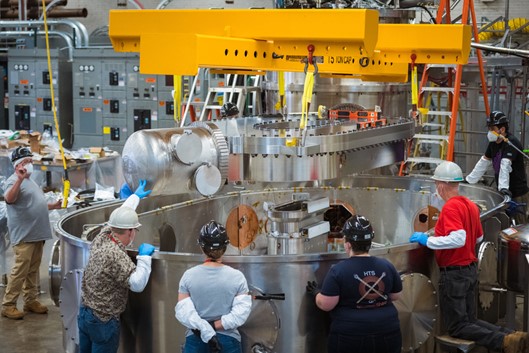
Bringing that new magnet concept to reality required three years of intensive work on design, establishing supply chains, and working out manufacturing methods for magnets that may eventually need to be produced by the thousands.
“We built a first-of-a-kind, superconducting magnet. It required a lot of work to create unique manufacturing processes and equipment. As a result, we are now well-prepared to ramp-up for SPARC production,” says Joy Dunn, head of operations for CFS. “We started with a physics model and a CAD design, and worked through lots of development and prototypes to turn a design on paper into this actual physical magnet.”
That entailed building manufacturing capabilities and testing facilities, including an iterative process with multiple suppliers of the superconducting tape, to help them reach the ability to produce material that met the needed specifications — and for which CFS is now overwhelmingly the world’s biggest user. They worked with two possible magnet designs in parallel, both of which ended up meeting the design requirements, she says. “It really came down to which one would revolutionize the way that we make superconducting magnets, and which one was easier to build.”
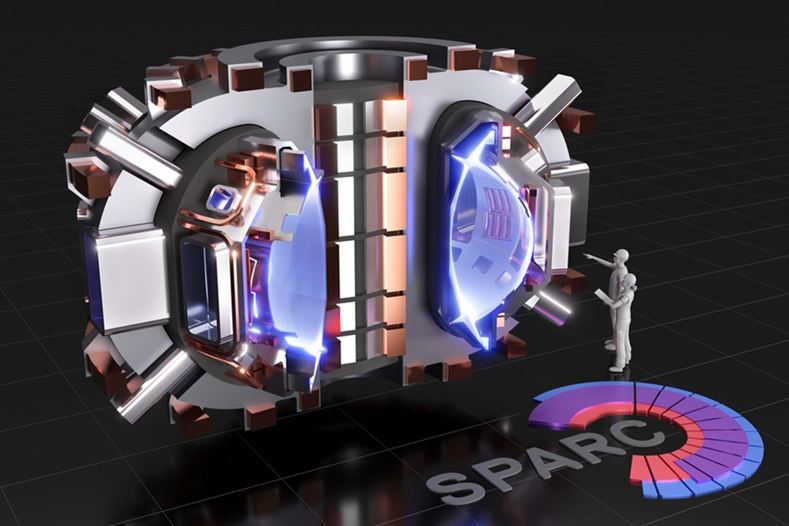
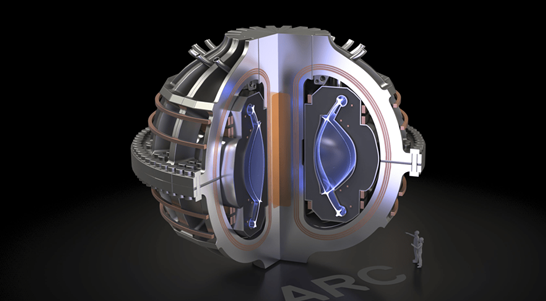
Founded in 2018, CFS was spun out of MIT and established to design and build fusion machines. Now its team includes experts in magnets, manufacturing and plasma physics collaborating with MIT on high-temperature superconducting magnet technology, using HTS magnets as a key enabler of compact fusion power plants that can be constructed faster and at lower cost.
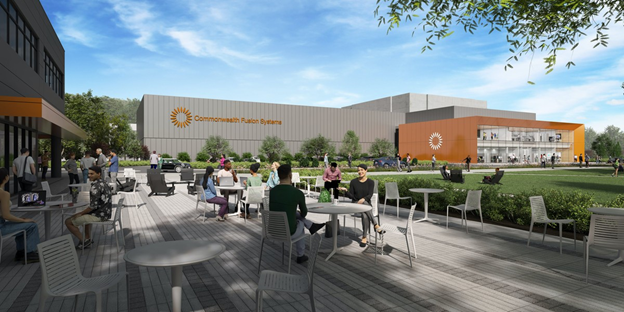
A year ago, CFS unveiled plans for building a 47-acre commercial fusion energy campus in Devens, Massachusetts, envisioned to become the birthplace of a commercial fusion energy industry and home to the SPARC compact fusion device. For more info, see www.cfs.energy and www.mit.edu.